Our Research Areas and Interests
Here you can find some of our main research areas we are working on. If you are interested in collaborating with us or have questions, please feel free to contact us.
Aeromaterials: Functionalities and applications well-beyond conventional aerogels
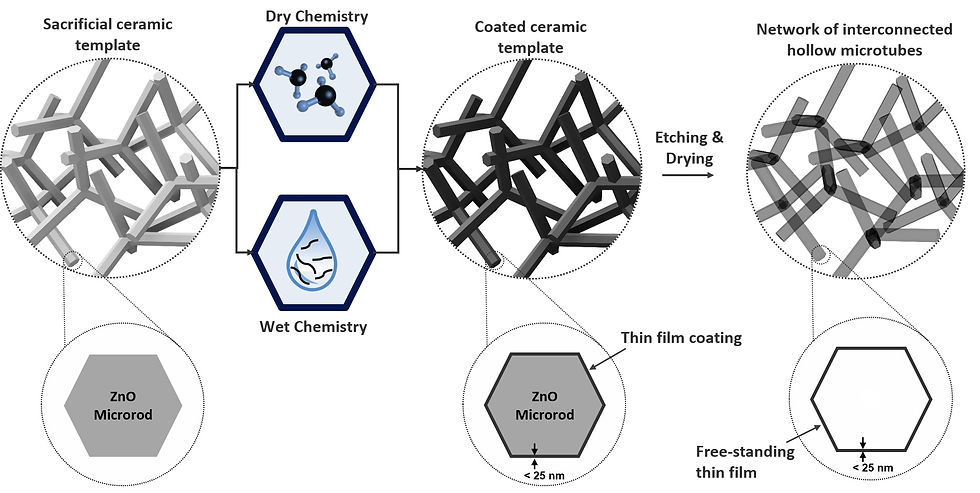
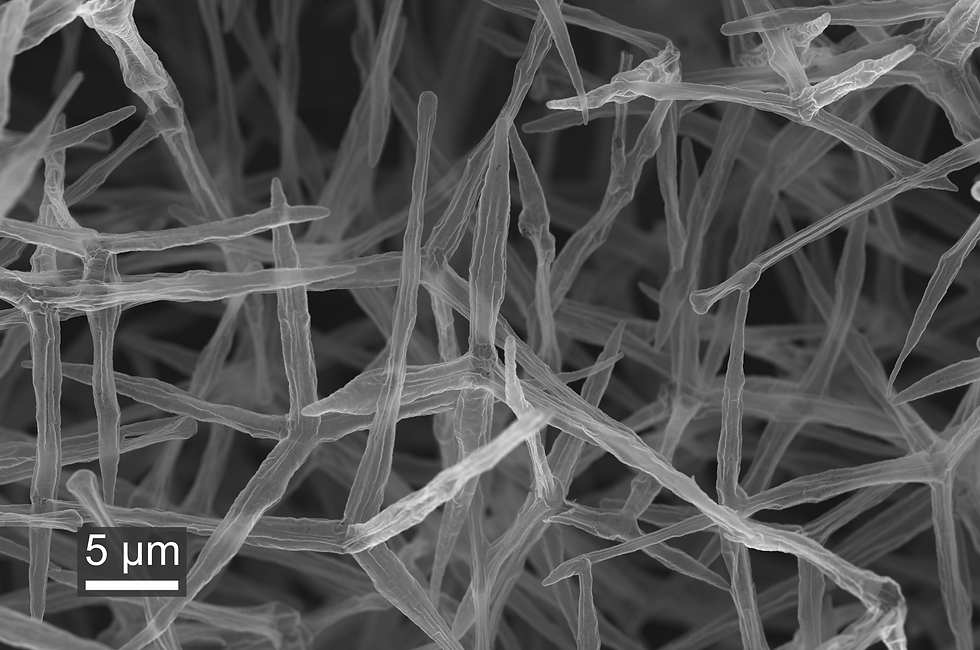

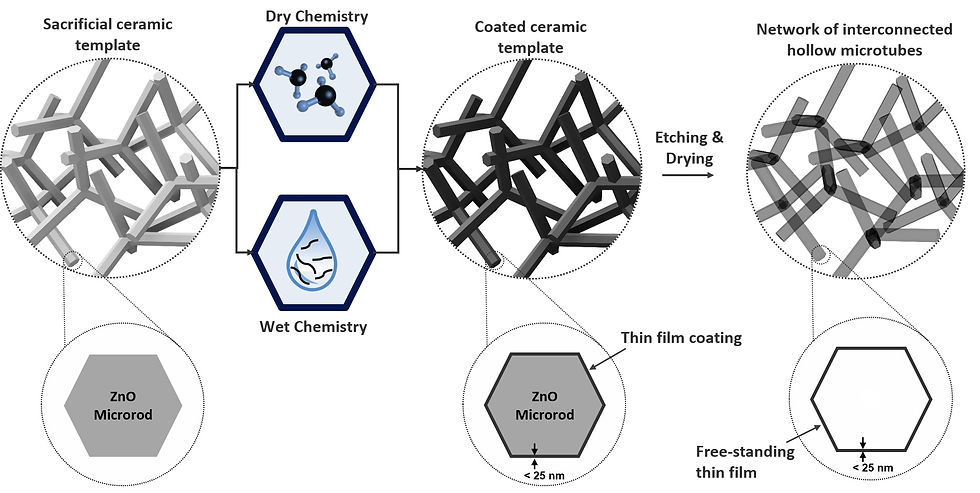
With a porosity of 99.99% our aeromaterials belong to the lightest materials on earth, with densities well below 1 mg/cm³. Based on their multi-scaled framework structure these aeromaterials are characterized by an extraordinary set of mechanical, thermal, electrical, as well as optical properties on the macroscale, which are defined by their nanoscale features. Thereby, our aeromaterials enable completely new functionalities well-beyond that of conventional aerogels. Based on our continuous research a broad range of different aeromaterials is available today, including aeromaterials based on carbon and related nanomaterials, such as Graphene, h-BN, MXenes and CNTs, aeromaterials based on semiconductors, such as GaN, aeromaterials from insulators, such as SiO2 as well as polymers. More information about these aeromaterials and their range of applications can be found in the following sections.
Aeromaterials: Innovative energy transducers
Hierarchical three-dimensional (3D) aerogels and foams of carbon and related nanomaterials have been shown to hold great promise as innovative energy transducer materials, e.g. for the conversion of light or electricity into heat, essential for a broad range of applications, such as the synthesis of solar fuels, thermal desalination and photoacoustic. However, the meso- and micropore structure of conventional aerogels fundamentally limits their conversion properties.
Electrically powered repeatable air explosions using microtubular graphene assemblies
We recently demonstrated that the extreme low heat capacity and high conductivity of graphene-based aeromaterials can be utilized to create electrically- powered and repeatable gas explosions. Controllable rapid expansion and activation of gases is important for a variety of applications, including combustion engines, thrusters, actuators, catalysis, and sensors. Typically, the activation of macroscopic gas volumes is based on ultra-fast chemical reactions, which require fuel and are irreversible. An “electrically powered explosion”, i.e., the rapid increase in temperature of a macroscopic relevant gas volume induced by an electrical power pulse, is a feasible repeatable and clean alternative, providing adaptable non-chemical power on demand. Till now, the fundamental problem was to find an efficient transducer material that converts electrical energy into an immediate temperature increase of a sufficient gas volume. To overcome these limitations, we developed electrically powered repeatable air explosions (EPRAE) based on free-standing graphene layers of nanoscale thickness in the form of microtubes that are interconnected to a macroscopic framework. These low-density and highly permeable graphene foams are characterized by heat capacities comparable to air. The EPRAE process facilitates cyclic heating of cm3-sized air volumes to several 100 °C for more than 100,000 cycles, heating rates beyond 300,000 K s−1 and repetition rates of several Hz. It enables pneumatic actuators with the highest observed output power densities (>40 kW kg−1) and strains ∼100%, as well as tunable microfluidic pumps, gas flowmeters, thermophones, and micro-thrusters.


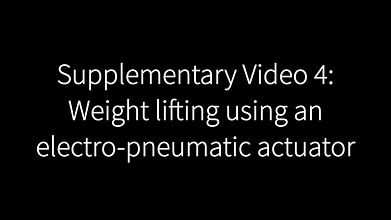
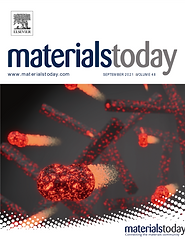
Artificial solid fogs for high-brightness laser light sources
Laser diodes are regarded as the next generation of ultra-efficient light sources, being able to produce more photons at high power densities than conventional light emitting diodes. Even though most state-of-the-art technologies are based on a blue LD pumping a white-light emitting phosphor, an all-laser wavelength mixing approach, e.g. a combination of three (RGB) or even four (RGBY) laser wavelengths would outperform the efficiency of any other known white-light source. However, in illumination applications, laser-based lighting systems still suffer from their monochromatic, low-divergent, and coherent nature, which demands a new generation of extremely efficient and versatile optical diffusers based on disordered nanostructured materials. Therefore, we have designed a macroscopically expanded, three-dimensional (3D) laser light diffuser based on a highly porous (>99.99%) nanoarchitecture, composed of interconnected hollow hexagonal boron nitride (h-BN) microtubes, with a wall thickness below 25 nm. The synthesized disordered and non-absorbing diffuser is based on a combination of feature sizes greater than, equal to, and well below the magnitude of the impinging wavelength. With densities close to that of air, these aeromaterials basically resemble an artificial solid fog, but with a defined hierarchical internal structure. This enables an isotropic 3D light distribution from energetic, highly directional, as well as coherent laser light, with speckle contrasts well below the human sensitivity limit.


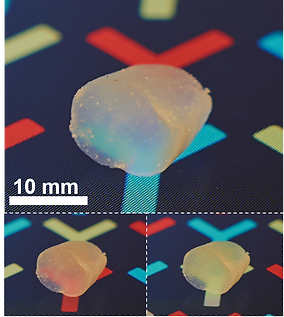

Aeromaterials: From passive to active air filtration systems
In the scope of a Spearhead project within the Graphene Flagship, called Aerograft, we are currently working together with Lufthansa Technik and other partners on the development of new air filter systems, capable of removing toxic compounds as well as biological threats from air streams. You can find out more here.
Conventional air filtration systems used in aviation are based on HEPA filters, which consist of fiber mats. These filters rely on the passive removal of air contaminants from the air stream passing through them, so they need to be replaced regularly. In the Aerograft spearhead project, we aim to replace these passive air filters with graphene foams that have a randomized framework structure. The graphene foams provide similar passive air filtration properties as passive HEPA filter systems, but they also enable us to turn the filter into an active system with new functionalities, such as monitoring environmental conditions, sterilizing pathogens, and self-cleaning.

Micro- and nano-engineered polymer-nanocomposites
Polymer-nanocomposites are materials that are composed of polymers and nanoparticles. The use of nanoparticles in these materials can enhance their mechanical, electrical, and thermal properties, resulting in a unique set of properties that make them useful in a wide range of applications, including drug release, soft robotics, flexible electronics, energy storage, and biomedicine. In order to further enhance the properties of such composites we develop innovative micro- and nano-engineering approaches that allow for precise control over their properties, and enable new functionalities. Thereby, we can customize the properties of a broad range of polymers, such as hydrogels and thermosets, to meet the specific requirements of different applications.
Multi-scaled drug release systems for local treatment therapies
Localized therapy approaches have emerged as an alternative drug administration route to overcome the limitations of systemic therapies, such as the crossing of the blood–brain barrier in the case of brain tumor treatment. For this, implantable drug delivery systems have been developed and extensively researched. However, to achieve an effective localized treatment, the release kinetics of drug delivery systems needs to be controlled in a defined manner, so that the concentration at the tumor site is within the therapeutic window. We developed a computationally supported reservoir-based drug delivery system towards patient-specific release kinetics. The fabrication of the drug release systems is based on a nano- and microengineering approach, by which a microchannel membrane is fabricated, surrounding a reservoir. By tailoring the drug delivery systems, in terms of membrane porosity, geometry, and drug concentration, the release profiles can be precisely adapted, with respect to the maximum concentration, release rate, and release time. The release is investigated using a model dye for varying parameters, leading to different distinct release profiles, with a maximum release of up to 60 days. Finally, a computational simulation, considering exemplary in vivo conditions (e.g., exchange of cerebrospinal fluid), is used to study the resulting drug release profiles, demonstrating the customizability of the system.

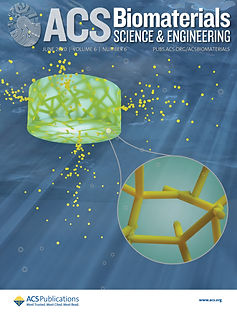
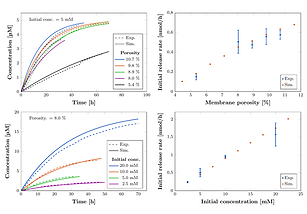
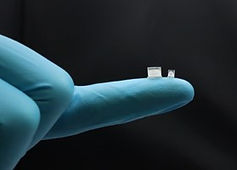
Multi-scaled conductive hydrogels
The fabrication of electrically conductive hydrogels is challenging as the introduction of an electrically conductive filler often changes mechanical hydrogel matrix properties. We have developed an innovative approach for the preparation of hydrogel composites with outstanding electrical conductivity at extremely low filler loadings. Therefore, exfoliated graphene and polyacrylamide are micro-engineered in the form of interpenetrating composites such that conductive graphene pathways pervade the hydrogel matrix similar to an artificial nervous system. This makes it possible to combine both the exceptional conductivity of exfoliated graphene and the adaptable mechanical properties of polyacrylamide. The demonstrated approach is highly versatile regarding porosity, filler material, as well as hydrogel system. The important difference to other approaches is that we keep the original properties of the matrix, while ensuring conductivity through graphene-coated microchannels. This novel approach of generating conductive hydrogels is very promising, with particular applications in the fields of bioelectronics and biohybrid robotics.



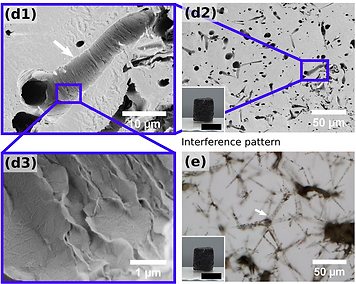
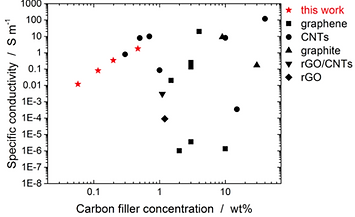
Micro- and nano-engineering of responsive hydrogels
Hydrogels that change volume in response to stimuli, known as stimuli-responsive hydrogels, are important in soft actuators research. One common type of stimuli-responsive hydrogel is thermoresponsive hydrogel, which changes volume in response to changes in temperature. Poly(N-isopropylacrylamide) (pNIPAM) is a common thermoresponsive hydrogel, but it has slow response rates and weak forces, which limits its use as an artificial muscle. We show that by incorporating interconnected microchannels into the pNIPAM hydrogel can significantly increase the response rate and volume change, while the stiffness of the swollen hydrogel remaines unchanged compared to the bulk hydrogel. The demonstrated concept can be easily adapted to other responsive hydrogels in order to enhance their properties and functionalities.

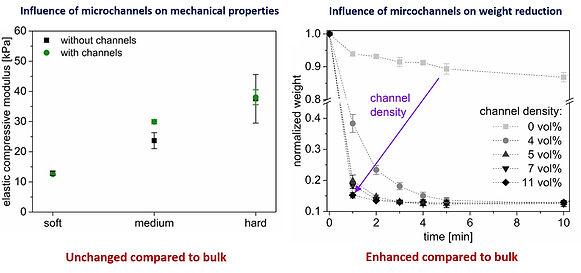